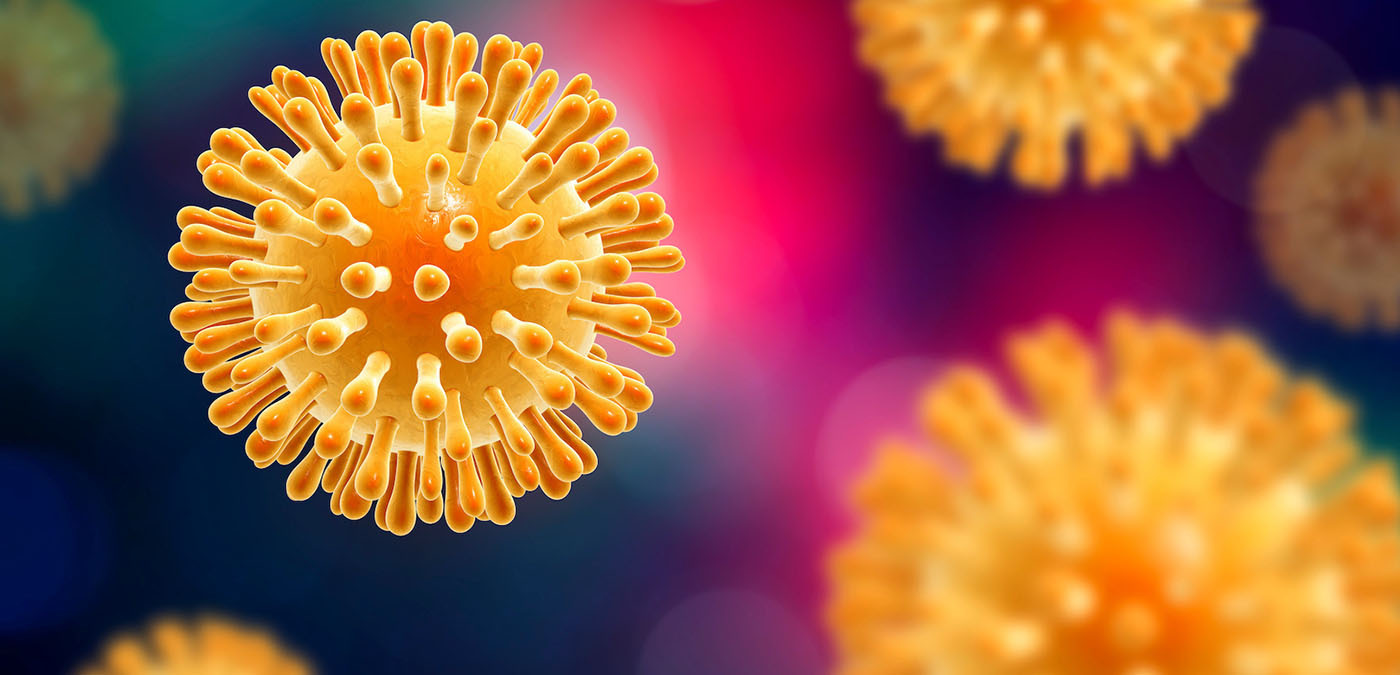
Gene therapy has brought new and exciting potential to the way we treat diseases. Yet, this approach requires delivering genetic material to specific target cells for a safe and efficacious treatment. Viral vectors are the favored vehicle used in gene therapy development and exhibit many advantages over non-viral methods, including high transduction efficiency, exquisite cell targeting, and a long history of pre-clinical and clinical use.
With several approved products and many more in the pipeline, the therapeutic applications of viral vectors continue to increase. In the following blog, we discuss the different types of commonly used viral vectors, critical considerations for construct design, and some current challenges in viral vector engineering.
What Are Viral Vectors?
Viral vectors are viruses engineered to deliver therapeutic genetic material into specific cells in the human body. This therapeutic payload can be a functional gene that replaces a non-functional mutant gene or a gene editing tool, such as the CRISPR-Cas system. Viral vectors are critical to the success of gene therapies as they can exert long-term expression of therapeutic genes, which can lead to potential cures for genetic and other diseases in many therapeutic areas.
The viruses currently used in gene therapies largely have been isolated from natural sources and retain their ability to infect cells. Over the past few decades, however, they have been engineered to overcome the various evolving challenges in gene therapy.
Commonly Used Viral Vectors
Viral vectors successfully used in the preclinical and clinical development of gene therapies include adenovirus (ADV) vectors, adeno-associated virus (AAV) vectors, and lentivirus vectors (LV). Choosing a suitable vector for your therapeutic application can be challenging; each has its advantages and disadvantages, with different expression levels, genomic integration strategies, transduction methods, packaging capacity, production process, and safety concerns.1
Adenovirus (ADV)
ADV is a non-enveloped DNA virus that commonly causes human upper respiratory tract infections.1 There are hundreds of human ADV genotypes, and the infection cycle has been heavily studied. The virus rarely causes severe infection in most healthy children or adults and is capable of transient transduction of nearly any mammalian cell type. Those infected carry lifelong immunity and express ADV-specific neutralizing antibodies.2
General features of ADVs:1
- High-level expression
- Capable of transient and long-term expression
- No genomic integration
- May be immunogenic
Lentivirus (LV)
LVs are complex retroviruses that are spherical, enveloped, and contain a single-stranded RNA genome. They constitute a genus of the retroviral family, the most infamous member of which is the human immunodeficiency virus (HIV).
Most LVs follow a typical infection cycle where the viral particle fuses with the cell membrane upon binding to cell surface receptors, and the genome is released into the cytoplasm. Following reverse transcription, the genome is integrated into the host genome non-randomly, favoring transcriptionally-active sites.3
General features of LVs:1
- High-level expression
- Capable of stable, long-term expression
- Integrates into genome
- Low immunogenicity
Adeno-associated viruses (AAV)
AAVs are small, non-enveloped viruses that carry single-stranded DNA. They don’t cause any known human disease, yet they have been a popular laboratory tool as it has a relatively simple genome and can be easily manipulated. Thousands of AAV variants have been classified into a few serotypes, with differential affinities to a range of cell surface receptors.4
General features of AAVs:1
- Moderate expression
- Capable of transient and long-term expression
- No genomic integration
- Low to moderate immunogenicity
Key Elements in Designing Viral Vector Constructs
Designing a viral vector construct is a crucial step in the early stages of the discovery and development of gene therapy. Several key factors must be considered when venturing down the path of using a viral vector construct for gene therapy development.
Viral Vector Type
Choose the appropriate viral vector type based on your specific application. As discussed above, different viral vectors can have various capacities for low or high expression over short or long periods. The size of the genetic payload that you want to deliver to a target cell will also shape which viral vector you choose for your application. For instance, “high-capacity” ADV vectors (e.g., third-generation ADVs) can accommodate 36 kb of foreign DNA cargo.5 In contrast, AAVs are severely restricted, only capable of accommodating 4.7 kb of cargo DNA. Lastly, the cellular target of the gene therapy can restrict the viral vector type you choose. Many different AAV serotypes and their cell specificity have been well-characterized. Several new targeting capabilities have been generated through rational design and directed evolution.6
Genetic Payload
The configuration of the genetic payload within the viral vector is primarily dictated by the viral vector selected. High-capacity ADV vectors, for example, contain inverted terminal repeat and packaging sequences, while the remainder of the vector consists of the transgenic expression cassette. However, some aspects of the transgene can impact the expression level of full-length protein and, hence, the safety and efficacy of a gene therapy. Codon optimization and removal of secondary structure are standard practices to address expression issues. Another element that has been gaining additional attention is the truncation of viral vectors during viral packaging, which can cause the production of capsids that have partial vector sequences and potentially lead to safety issues.7 Recently, this issue has been called out in FDA guidance as a critical quality attribute that needs to be analyzed in pre-clinical and clinical development.8 Eliminating specific sequences associated with the formation of secondary structures can help reduce genome truncation and eliminate these production impurities.
Promoter Selection and Expression Cassettes
Promoter selection is an essential aspect of vector design, particularly with AAV-based vectors. Certain AAV serotypes can infect multiple tissues and cell types. Thus, to avoid off-target expression, transgene expression must be controlled by promoters that are only expressed in target cell types at a level necessary for efficacy.
Immunogenicity and Other Safety Issues
Given the tumultuous history of viral vectors, safety issues are front and center in the current age of gene therapy development.9 Much effort has been devoted to reducing immunogenicity, as the tragic case of Jesse Geisinger resulted from an immune response due to an ADV-based gene therapy. Thankfully, third-generation ADVs have reduced immunogenicity compared to older ADV technologies.
AAV vector design has focused on removing factors that can induce innate immune responses. Unmethylated CpG dinucleotides, for instance, are known activators of Toll-like receptor 9 (TLR9), and the removal of CpG sequences from vectors can help reduce immune activation.10 In addition, including microRNAs in constructs that target the expression of T-cell activating proteins can also reduce innate immune activation.11
Challenges and Solutions in Viral Vector Development
ADV, AAV, and LV vectors are all under investigation for the delivery of gene therapies, and each has appropriate use cases depending on the expression level required and the target tissue/cell type.
Yet their selection must also be balanced with the current safety challenges being faced for each vector type. One core issue for LV vectors is their inappropriate insertion into the genome, which can lead to oncogenesis.12 To remedy this, non-integrating LV vectors are being investigated.
For ADV and AAV vectors, managing immunogenicity continues to be an issue. Many people have pre-existing immunity against ADV vectors, and treatment with an ADV-based gene therapy would lead to a robust adaptive immune response, limiting efficacy and raising safety concerns. These issues are being solved by investigating “rarer” serotypes or ADV vectors isolated from animals.13 AAV vectors face innate immune sensors (e.g., TLR9) as a significant barrier. In particular, cytosolic nucleic acid sensors can induce a type I interferon response, which limits the efficacy of AAV-based gene therapies.14
Due to impurities in AAV preparations, dosing is another problem. Dealing with this and other AAV biomanufacturing scalability issues requires a multi-faceted approach requiring tight collaboration between drug developers and regulatory agencies. AI tools have helped address some of the production issues with AAV vectors by predicting problematic sequences during the construct design phase.15
The Future of Viral Vector Construct Design
While viral vector-based gene therapies hold significant promise, some developability and manufacturability barriers remain. However, when challenging problems arise, particularly those related to patient populations with limited treatment options, interdisciplinary approaches with innovative solutions tend to emerge. With new viral vector characterization, manufacturing, and computational tools, multi-faceted teams are creating ways to design more effective and manufacturable gene therapy constructs.
At Form Bio, we’ve recently launched FORMsightAI, an AI solution that supercharges AAV gene therapy development. Previously, assessing this was a trial-and-error process that required a heavy time and cost investment. Our platform is helping assess the quality of AAV bioreactor runs and predicting manufacturing outputs. Together, these AI-based solutions are accelerating gene therapy.
To learn more about how our AI models work, read our biological validation studies
Download nowReferences
- Bulcha JT, Wang Y, Ma H, Tai PWL, Gao G. Viral vector platforms within the gene therapy landscape. Signal Transduct Target Ther. 2021;6(1):1-24.
- Barouch DH, Kik SV, Weverling GJ, et al. International seroepidemiology of adenovirus serotypes 5, 26, 35, and 48 in pediatric and adult populations. Vaccine. 2011;29(32):5203-5209.
- Ciuffi A. Mechanisms governing lentivirus integration site selection. Curr Gene Ther. 2008;8(6):419-429.
- Calcedo R, Vandenberghe LH, Gao G, Lin J, Wilson JM. Worldwide epidemiology of neutralizing antibodies to adeno-associated viruses. J Infect Dis. 2009;199(3):381-390.
- Alba R, Bosch A, Chillon M. Gutless adenovirus: last-generation adenovirus for gene therapy. Gene Ther. 2005;12 Suppl 1:S18-27.
- Yang YS, Xie J, Wang D, et al. Bone-targeting AAV-mediated silencing of Schnurri-3 prevents bone loss in osteoporosis. Nat Commun. 2019;10:2958.
- Developing Machine Learning Powered Solutions for Cell and Gene Therapy Candidate Validation. Accessed September 12, 2023.
- FDA Clinical Trial Guidance For AAV Gene Therapy | Form Bio. Published March 15. 2023. Accessed September 13, 2023.
- Raper SE, Chirmule N, Lee FS, et al. Fatal systemic inflammatory response syndrome in a ornithine transcarbamylase deficient patient following adenoviral gene transfer. Mol Genet Metab. 2003;80(1-2):148-158.
- Faust SM, Bell P, Cutler BJ, et al. CpG-depleted adeno-associated virus vectors evade immune detection. J Clin Invest. 2013;123(7):2994-3001.
- Brown BD, Venneri MA, Zingale A, Sergi LS, Naldini L. Endogenous microRNA regulation suppresses transgene expression in hematopoietic lineages and enables stable gene transfer. Nat Med. 2006;12(5):585-591.
- Hacein-Bey-Abina S, Garrigue A, Wang GP, et al. Insertional oncogenesis in 4 patients after retrovirus-mediated gene therapy of SCID-X1. J Clin Invest. 2008;118(9):3132-3142.
- Chen H, Xiang ZQ, Li Y, et al. Adenovirus-based vaccines: comparison of vectors from three species of adenoviridae. J Virol. 2010;84(20):10522-10532.
- Chandler LC, Barnard AR, Caddy SL, et al. Enhancement of adeno-associated virus-mediated gene therapy using hydroxychloroquine in murine and human tissues. Mol Ther Methods Clin Dev. 2019;14:77-89.
- The Emerging Role of AI in The Future of Gene Therapy. Published July 11, 2023. Accessed September 13, 2023.
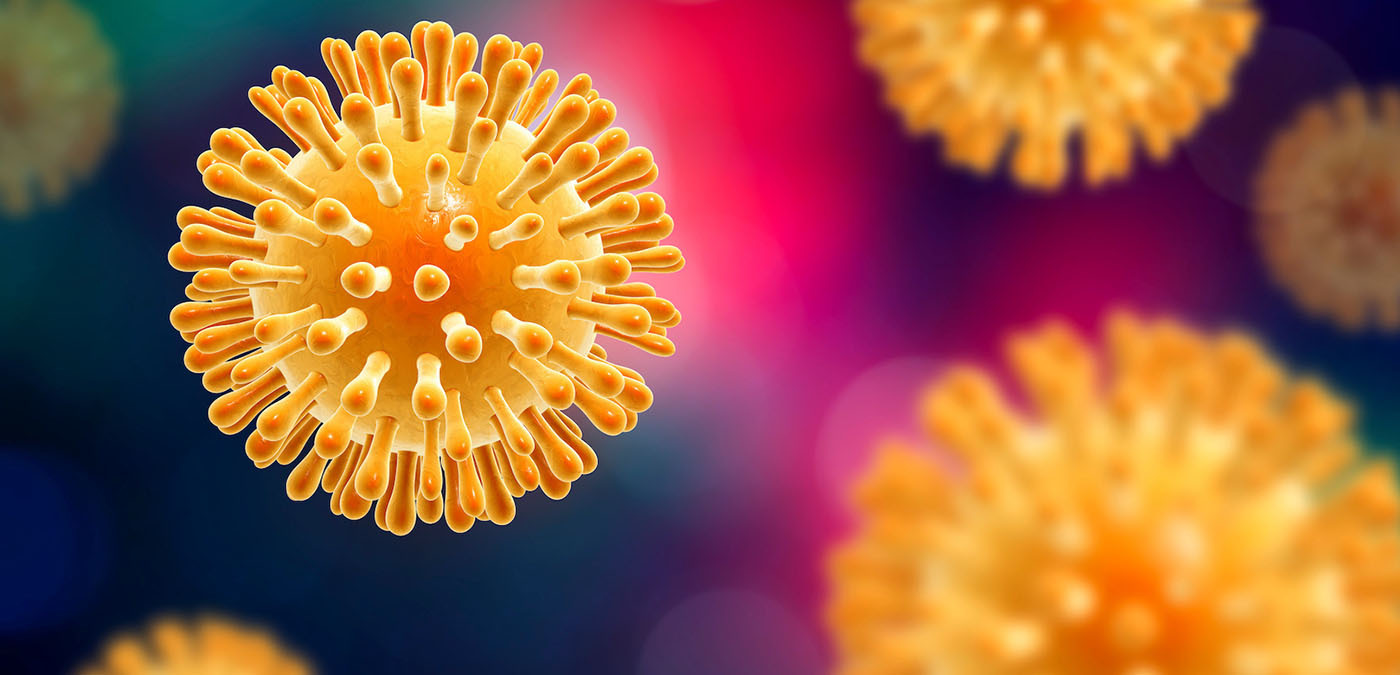